I have great respect for Dr. Robert Naviaux with his vast knowledge on mitochondrial function and would urge you to watch his video on Mitochondria and Autism. Even if you do not have an interest or understanding of autism it is a great video to watch to understand the role of the mitochondria in cellular function and health.
About 1500 genes are needed to make mitochondria. Only 13 proteins are encoded for by the mitochondrial DNA while the others are dependent on tissue-specific gene expression depending on what organ or cell needs to be made.
Mitochondrial dysfunction (not disease) occurs when there’s a mitochondrial shift in response to a threat (toxin, infection, pollution, metals, etc.) and is a regulatory mechanism to ensure the cell’s survival. Mitochondrial markers on organic acid tests (OAT) are often treated by introducing mitochondrial nutrients. But maybe the focus should not be on plying the mitochondria with more nutrients and flog a dead horse, but rather to take notice of any possible threat, identify it by looking for infections or toxicity, and then remove it.
After all, our mitochondria is just the first sensor to detect biochemical changes in our cells before disease processes set in, and we need to take notice of this.
Mitochondria is NOT just energy production
Energy production is a secondary function of the mitochondria. Other pathways in the body can take over energy production if the mitochondria is unable to. It’s not ideal for optimal health, but this process can move forward independent of the mitochondria and krebs cycle through anaerobic metabolism that does not require oxygen. This is usually the case when mitochondria are unhealthy or compromised due to lack of nutrients, lack of oxygen or infections. During these times glycolysis will become the dominant energy production pathway which takes place inside the cell but outside of the mitochondria. Lactate or lactic acid is produced in the process.
We discuss the different energy production pathways and how efficient they are in the article Mitochondria – NADH and ATP Production via Different Pathways.
Lactic acid is responsible for the muscle fatigue experienced after intense exercise. Oxygen and energy run low and the biochemistry of the athlete shifts towards glycolysis to maintain energy production in a low oxygen environment, producing lactic acid as a byproduct of metabolism. Typical symptoms of chronically elevated lactic acid include:
- Fatigue
- Muscle fatigue
- Body aches and pains
- Brain fog
These are common symptoms reported by those with chronic candida infections which would make sense since candida thrives in low oxygen environments and manipulate our biochemistry by upregulating glycolysis.
Mitochondria as a Communicator and Adaptor
This is the main and primary role of the mitochondria.
They constantly measure the cellular environment, temperature, pH, oxygen, nutrient status, inflammation, etc. and communicate to surrounding cells, immune cells and enzymes. Imagine them being like security guards patrolling a building. This allows the body and cells to adapt to the changing environment in order to survive.
Mitochondrial markers (which can be tested for in an organic acid test) are often the first biochemical markers to change when homeostasis is disrupted.
Mitochondria in a Happy Environment
When mitochondria is in a good place with good nutrition, they produce small molecules containing ATP (adenosine triphosphate), alpha-ketoglutarate (AKG), succinate, etc. that gets sent to the nucleus and then outside the cell where it creates a ‘halo’ around the cell. This is a kind of signal to neighbouring cells that reads ‘I am well’.
90% of catabolic reactions (anaplerosis) and 70% of anabolic reactions (cataplerosis) pass through the mitochondria at some point. See how you cannot lose weight, build muscle or repair your body without good mitochondrial function?
The Concept of Cataplerosis and Anaplerosis in Weight Loss
Mitochondrial Stress & Apoptosis
When cells are put under increasing amounts of stress through changes in pH, temperature changes (burns, hypothermia), nutritional deprivation (lack of food or overeating) or oxygen deprivation (stroke) mitocondria stop producing this ‘halo’ around the cell and is an early alarm system that things are not going so well.
The mitochondria is the first to sense that something is ‘off’ due to a shift in electrons, and mitochondrial dysfunction can be measured before actual disease even sets in. If the stress continues mitochondria will change from a filamental form to a more spherical or fragmented form. This is the shape of mitochondria often seen before apoptosis (where cells commit suicide).
After apoptosis the inner phosphatidylserine part of the mitochondrial cell membrane flips to the outside of the cell membrane. Fragmented mitochondrial DNA and inflammatory peptides are released from the damaged mitochondria into the cellular environment triggering inflammation and immune over-reactivity. Methionine on the DNA is recognised as foreign by the immune system triggering anti-DNAse antibody formation, and phagocytes as part of the immune response are recruited to the area to clean up. Neutrophils are also recruited through this chemotactic response.
In the end, if the mitochondria dies, the cell dies.
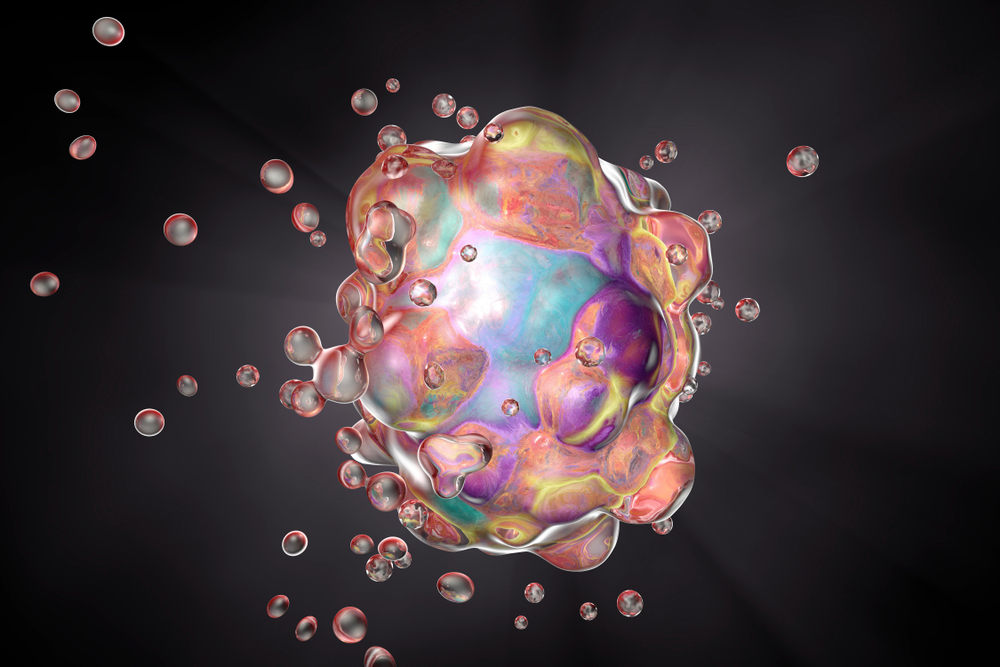
Injured cells
During injury (stroke, sprained ankle, cuts) oxygen supply is cut off to the area which means cellular mitochondria around the injured area can only produce very limited ATP or energy via the oxygen-dependent Krebs Cycle.
This is a problem as repair mechanisms require lots of ATP. So, as a short-term quick-fix solution metabolism switches from anaerobic to aerobic glycolysis using the short cut rout to convert glucose into energy for cell survival. The surrounding ATP generated can also be imported into the damaged mitochondria and start turning ATP synthase in the opposite direction to pump protons back into the inner membrane space in order to maintain the inner mitochondrial membrane potential. This maintenance of the membrane potential is the only way the mitochondria can tell the nucleus that they need supplies and the only way the supplies (amino acids and other building blocks) can find the mitochondria for delivery.
If there is no charge, there is no supplies and thus no repair.
The inner mitochondrial membrane is also vital for producing orotic acid, the substrate for UMP (uridine monophosphate). UMP is needed for DNA and RNA synthesis and activating glycogen metabolism.
Acute Injury vs Chronic Stress
During acute injury mitochondria will reduce their activity, almost going into hibernation, letting glycolysis take over as an emergency supply for ATP. This provides quick energy with minimal effort and less free radical production.
When the danger is gone and the cells need to start healing, mitochondria will get out of hibernation and start to increase their activity. Even though glycolysis provides quick energy, oxidative phosphorylation and the krebs cycle inside the mitochondria produce far more energy for healing and repair.
This is the natural order. Glycolysis is a temporary ‘fix’ but is not meant for long-term reliance. Unfortunately this is often what happens with chronic stress or injury and is also a common biochemical occurrence in autism.
The cells become stuck in glycolysis.
Sometimes this is because the injury or threat persists and hasn’t been dealt with making the mitochondria stay in hibernation. This is where the whole mantra of ‘treating the root cause’ has come from.
However, the threat may have already been dealt with but cells are reluctant to move out of this phase. This is often seen in autism where infections and toxins have been dealt with, improvements in symptoms have been observed, but the child hasn’t fully recovered. This has a lot to do with purinergic signalling which is a discussion for another day.
The point being that if cells become chronically stuck in glycolysis then they cannot fully repair or recover which is what we typically see in chronic disease.
Mitochondrial Downregulation as a Protective Mechanism
The electron transport chain (ETC) uses oxygen during cellular respiration to produce ATP/energy from glucose but also leaves a lot of free radicals like superoxide behind in the process which can be very damaging to the mitochondria without antioxidants to neutralize them. A balanced redox state where there is enough antioxidants (from a healthy diet) to neutralize these free radicals is needed to keep the cells healthy and working.
If the mitochondria senses that the free radicals are starting to outweigh the neutralizing antioxidants it will slow down in an effort to protect itself from damage. This is the main reason why very sick people feel worse when they take nutrients that stimulate their mitochondria. As previously stated it’s like flogging a dead horse. Redox balance needs to be restored first. It also shows how a diet high in sugar and low in antioxidants (fruit and vegetables) contribute to this unbalanced redox state.
The next question is where do all these free radicals come from?
Some from mitochondrial production (as just discussed), but also from upregulated phase 1 liver detoxification or the P450 cytochrome system. During phase 1 liver detoxification molecules are oxidized into active free radicals. Phase 2 then has to step up and produce another molecule such as glutathione, amino acids, or sulphur to bind to the free radical and ‘neutralize’ it, making it less active and water-soluble. Now it can be excreted out of the body.
When phase 1 is either working too fast for phase 2 to keep up as seen in infections and toxin exposure, or phase 2 is not supported due to methylation or sulfuration issues, or even worse both, excessive amounts of free radicals will force the mitochondria to slow down.
This is more commonly recognized as chronic fatigue syndrome (CFS).
Mitochondria and Disease
Many conditions such as macular degeneration, retinal detachment, Leu Garicks disease, ALS, neurological diseases are all being contributed to mitochondrial dysfunction, but in reality all disease has an element of mitochondrial dysfunction.
What causes the mitochondria to become dysfunctional?
- Nutritional deficiencies (inside the cell)
- Excess carbohydrates and fructose in the diet
- Infections, dysbiosis and mycotoxins
- Acetylaldehyde from alcohol or yeast infections
- Quinones from hormone and neurotransmitter detoxification
- Xenobiotics and environmental toxins (POPs)
- Antibiotics, medications, recreational drugs
- Sulfites and hydrogen sulphide from CBS (cystathionine-beta-synthase) inhibition and gut dysbiosis
- Poor genes around detoxification – P450, SOD (superoxide dismutase), GS (glutamine synthetase) – and their expression
Everything listed here relates back to diet, infections and our environment.
As we get sicker and older, we produce less and less mitochondria, or start losing them through apoptosis as ageing cells start to die. Since the mitochondria is responsible for keeping an eye on things and sounding the alarm when things go wrong, our bodies become less efficient in doing this job which makes us more vulnerable.
Methylation and CDR
The Cell Danger Response (CDR) triggers the decarboxylation of SAMe (S-adenosyl-L-methionine) for polyamine synthesis typically seen in auto-immune disease which creates a SAMe deficiency and thus compromises the methylation cycle. SAMe deficiencies reduce phospholipid synthesis needed for healthy cell membrane production which results in a stiffening of cell membranes regardless of the type of fats you consume or your cholesterol levels. This will greatly affect nutrient transport and communication between cells.
But it doesn’t just affect SAMe. CDR also acts like an MTHFR (methylene tetrahydrofolate reductase) snip by shifting folate metabolism towards folinic acid away from 5-MTHF (methyl tetrahydrofolate) for DNA repair as opposed to SAMe production.
Once the CDR is over, methylation returns to normal as SAMe stops being decarboxylated.
Mitochondria and Infections
Remember that NADPH is created from the PPP (Pentose Phosphate Pathway) for various functions which you can read more about in Mitochondria – NADH and ATP Production via Different Pathways.
One of those functions is to create SO (Superoxide) for fighting pathogens. Those with chronic infections such as Lyme, candida or other viral and bacterial infections often experience a shift in NADPH away from glutathione and cholesterol production towards superoxide production instead. In my opinion there is a continual shift as the body weighs the pro’s and con’s between different actions. If it deems fighting microbes more important, it will be to the detriment of making cholesterol for bile and cell membrane repair, and less glutathione to protect against DNA damage and facilitate heavy metal detoxification. In the process a lot of free radicals are produced that causes further damage to the cells. However, if we just focus on reducing superoxide we may be interfering with the body’s attempt to fight off chronic infections. This could be a possible reason why some people with chronic infections feel worse when they take antioxidant supplements.
If heavy metals are the focus then maybe NADPH will shift more towards glutathione regeneration with less emphasis on superoxide.
Either way, the PPP is upregulated during oxidative stress and mitochondrial stress.
But it’s not just the PPP that can be upregulated. CBS can also be upregulated regardless of genetic snips. This may further impair mitochondrial function through the production of hydrogen sulphide, ammonia, oxalates, histamine, cortisol, etc.
If SUOX (sulfite oxidase) is down-regulated it will add to the problem with lower sulfate excretion and more sulfite accumulation which often contribute to sulfur sensitivities.
Cortisol and CDR
Whenever we experience stress the hypothalamus releases adrenocorticotropic hormone (ACTH) from the pituitary gland which binds to MC2-R receptors on cells in the adrenal cortex. Stress can be defined as emotional stress, starvation or inflammation as all of these triggers will stimulate this response.
This signals for StAR (Steroidogenic Acute Regulatory Protein) to import cholesterol into the mitochondria where it is converted in a 2-step process first into 17-OH-pregnenolone and then 11-deoxycortisol inside the endoplasmic reticulum (ER). 11-deoxycortisol is returned from the ER back to the mitochondria where it is transformed into its final end-product, cortisol.
Cortisol can freely cross the cell membranes and enter the bloodstream. Purinergic signalling stimulates cortisol synthesis during the CDR which would shunt cholesterol all the way down cortisol production away from other steroid hormones and cell membrane repair.
Why is this important?
Because NADPH is either being used for superoxide against infections or glutathione regeneration to remove heavy metals. Often the focus is not on cholesterol synthesis, and you often see this in very sick people where they have low cholesterol, low hormones, low neurotransmitters, and either high cortisol in the early stages or low cortisol when adrenal fatigue sets in.
Low Blood Sugar and CDR
The drop in oxygen experienced during the CDR triggers an upregulation of the GLUT (glucose transporter) enzymes.
GLUT transporters allow glucose entry from the bloodstream into the cells for various reasons such as glycolysis, NADPH production and triggering cellular proliferation for repair. This is why we often see hypoglycaemia or blood sugar problems in those with chronic health issues.
This pattern is also seen in cancer.
Purinergic Receptors and getting out of the Cell Danger Response (CDR)
Dr. Robert Naviaux came up with the theory that the purinergic receptors need to be rebooted in order to get out of a stuck CDR. Suramin is one medication that can reboot all 19 purinergic receptors, but more research is being done in this area at the time of writing.
Innate Immunity
The mitochondria controls the first steps in innate immunity.
Mitochondrial anti-viral sensing proteins bind to the double-stranded RNA and DNA. This coordinates a phosphorylation reaction that activates interferon-response-factors 3 and 7. These go to the nucleus and coordinates the interferon response to that cell.
It also amplifies the NF-κB response. NF-κB (nuclear factor kappa-light-chain-enhancer of activated B cells) is a protein complex that controls transcription of DNA, cytokine production and cell survival.
mtNOS & Nitric Oxide
Mitochondrial Nitric Oxide Synthase (mtNOS) is a newly discovered form of NOS (nitric oxide synthase) that is calcium-dependent and plays a role in the regulation of respiration.
We discuss calcium regulation and mitochondrial dysfunction in more depth in the article The Importance of Calcium in Mitochondrial Function and Regeneration.
The mitochondria has an interactive role with calcium channels as it stores calcium which is very important for respiration and subsequent energy production.
Mitochondria – What Happens When You Drink Alcohol
Mitochondrial Dysfunction – What Does It Mean For You?
References:
Role of exhaled nitric oxide in asthma
Dr. Robert Naviaux’s (M.D.Ph.D.) work
Dr. Ben Lynch N.D. from SHEICON15
Picard, et. al. Mitochondrial functions modulate neuroendocrine, metabolic, inflammatory, and transcriptional responses to acute psychological stress. Proc. Natl. Acad. Sci. USA. 2015 Dec. 1;112(48)
I love it. Great work
Thanks Petra 🙂